Navigating Hydrogen
How does the future of hydrogen look under net zero targets?
Scientific Properties of Hydrogen
Hydrogen, symbolized as H, is the lightest and most abundant element in the universe. With an atomic number of 1, it sits at the top of the periodic table. Hydrogen is a diatomic molecule under standard conditions, existing as H₂ gas, and is colourless, odourless, and non-toxic. It is the simplest atom, consisting of one proton and one electron, making it a fundamental building block of matter.
Hydrogen is unique due to its isotopes: protium (¹H), deuterium (²H, or heavy hydrogen), and tritium (³H), the latter being radioactive. Its low density and high reactivity mean it rarely exists freely in nature, instead forming compounds like water (H₂O) and hydrocarbons. Hydrogen’s high energy content per unit weight has made it an attractive candidate for clean energy solutions. It also serves as a critical reactant in processes like the Haber-Bosch method for ammonia production.
Uses and Future Applications
Hydrogen plays an integral role in various industries due to its versatility. Global demand for hydrogen in 2023 was 97 million tonnes (Mt) according to the IEA Global Hydrogen Review 2024. The primary uses include:
Refining – 43Mt (44% of demand): Hydrogen is used to break down large, heavy hydrocarbon molecules into lighter, more valuable products like diesel, jet fuel, and gasoline and is useful to remove sulphur from fuels in the oil refining process.
Industrial Uses 54 Mt – (55.7% of demand): Hydrogens industrial uses can be broken up into ammonia (60%), where hydrogen is a key feedstock for producing ammonia, as well as for methanol production (30%), and lastly for direct reduced iron (DRI) in the iron and steel sector (10% of industrial demand). Hydrogen’s use in DRI is by refining and reducing metal ores (removing oxygen content) in its solid state without melting, -rather than using coke/metallurgical coal in blast furnaces.
Other uses: Hydrogen is also used for energy production in fuel cells to generate electricity, emitting only water vapor, making it a cornerstone of the green energy transition. Hydrogen also serves as a rocket propellant in aerospace due to its high energy density.
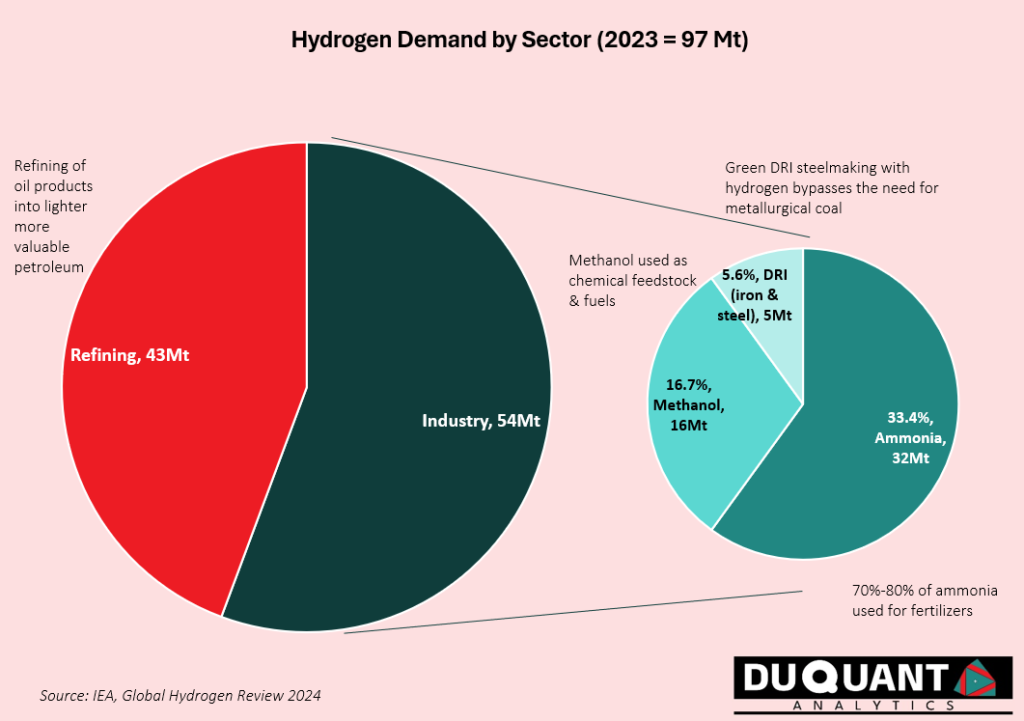
The global demand for hydrogen is expected to rise significantly (from ~100Mt in 2024 to ~150Mt by 2023 under NZE scenario) due to its potential to decarbonize sectors like transportation, heavy industry, and power generation. “Green hydrogen,” produced via water electrolysis powered by renewable energy, is particularly promising, and currently a tiny market. Countries and corporations are investing billions to scale up production and it’s projected that up to 45% of the ~150Mt hydrogen by 2030 will be from low-emission sources (currently <5%). Growth opportunities also lie in hydrogen storage and distribution technologies, vital for enabling its widespread adoption, particularly in the transportation sector. Currently Korea, China, Japan, USA and Europe are leading the fuel cell EV market, at around 90,000 vehicles global in 2024 (small vs 1.5 billion vehicles total). Investors are drawn to hydrogen’s role in achieving net-zero emissions, making it a long-term growth market from its current small role in the global energy mix. Long term catalysts for the hydrogen sector will be cheap and excess electricity supply, which could come from an abundance (over investment) of supply from renewables such as wind and solar.
Largest Sources and Producers of Hydrogen
Globally, in 2023 around 97 million tonnes (Mt) of Hydrogen (H2) was produced. China accounted for around 30% of globally supply, followed by USA (~14%), Middle East (~14%) and India (~9%). Global production is estimated to reach 100Mt by the end of 2024, and under the Net Zero emissions by 2050 scenario, the hydrogen market is expected to reach close to 150Mtpa by 2030 (8.4% CAGR from present), and 45% of which is low-emission hydrogen (currently <5%).
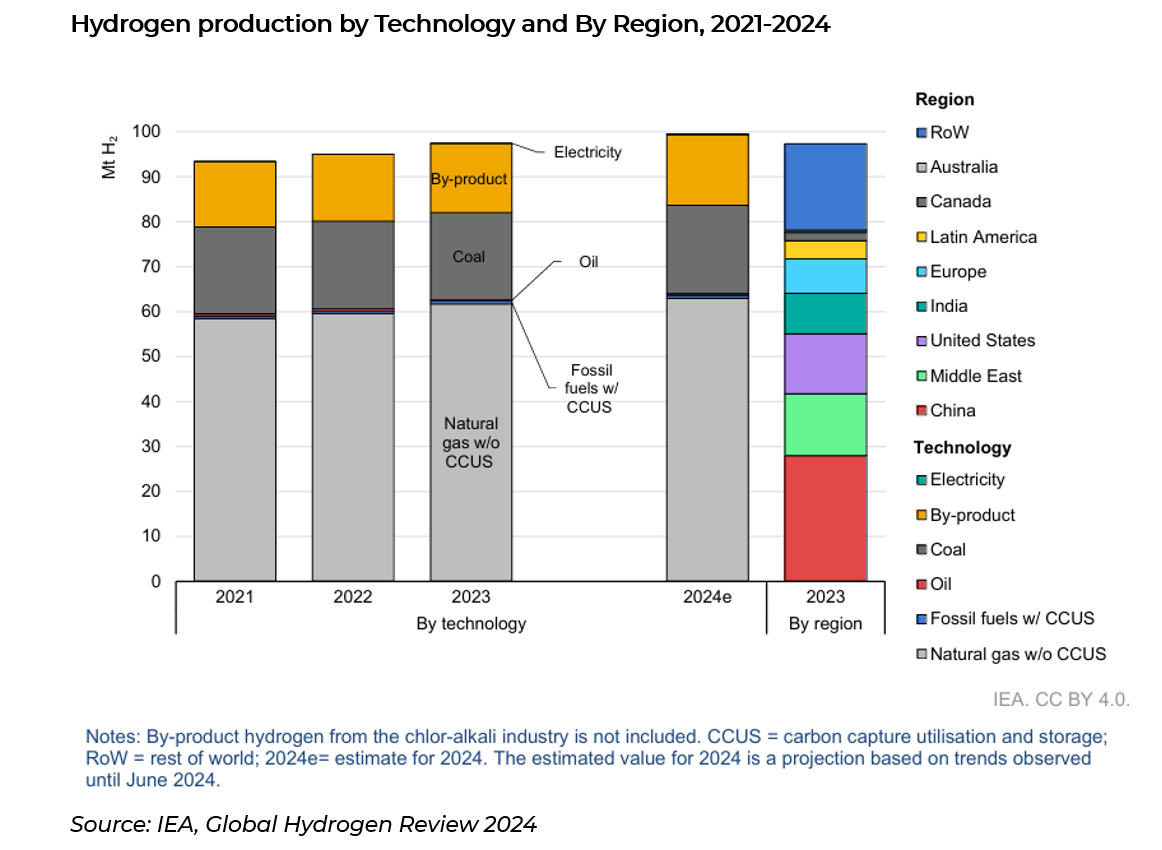
Low emission hydrogen is still an extremely small market, making up around 5% of total hydrogen supply. Low emission hydrogen is produced in two ways, one of them called blue hydrogen from fossil fuels (natural gas), and the other being green hydrogen from electrolysis (using electricity).
Blue hydrogen is produced through a process called steam methane reforming (SMR), where natural gas (methane) is reacted with steam under high pressure and temperature to produce hydrogen and carbon dioxide. The hydrogen is then purified for use, while the CO₂ is typically captured and stored underground through carbon capture and storage (CCS) technology, which mitigates emissions. Despite being cleaner than grey hydrogen (produced without CCS), blue hydrogen still relies on fossil fuels, making it less sustainable than green hydrogen.
Green hydrogen is produced using electrolysis, a process that splits water (H₂O) into hydrogen (H₂) and oxygen (O₂) using electricity. For green hydrogen, the electricity must come from renewable sources such as solar, wind, or hydroelectric power, ensuring zero carbon emissions during production. Unlike blue hydrogen, green hydrogen does not rely on fossil fuels, making it a key technology for achieving carbon-neutral energy systems and supporting a clean hydrogen economy.
The downfall and critique of green hydrogen produced through electrolysis is that it typically has an energy efficiency of around 60-70%, meaning 30-40% of the input electricity is lost as heat or in the conversion process. This inefficiency arises from the energy required to split water molecules and from losses in the electrolyser system. Additionally, further energy losses occur if the hydrogen is compressed, liquefied, or transported, reducing the overall efficiency of the system to around 50-60% from renewable electricity to usable hydrogen energy.
Green hydrogen production inefficiencies could avoid this waste if production is aligned with periods of excess electricity generation from renewable sources, such as during peak solar production or strong winds, thus preventing renewable energy curtailment by converting recess electricity into hydrogen gas which could be used in industry, and thus is a practical form of energy storage that is not in the form of batteries.
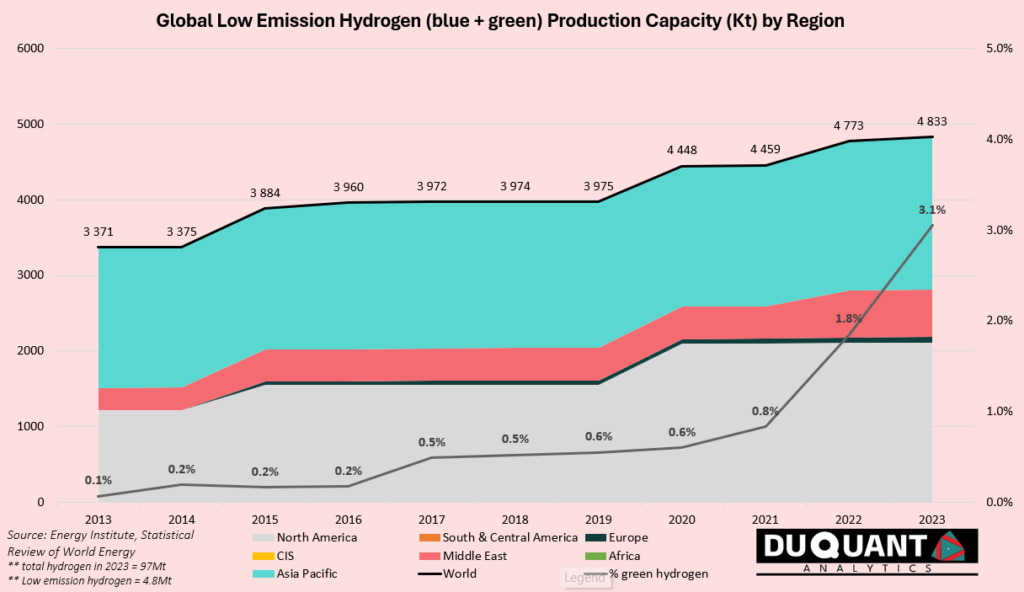
The Remarkable History of Hydrogen
The story of hydrogen dates back to ancient philosophy, but its formal discovery began in the 16th century with Paracelsus, who observed bubbles forming during the reaction of metals with acids. However, it was Henry Cavendish in 1766 who first identified hydrogen as a distinct substance, calling it “inflammable air.” Cavendish also demonstrated that water is a compound of hydrogen and oxygen, a groundbreaking revelation at the time.
Hydrogen’s name, derived from the Greek words for “water” and “generator,” was coined by Antoine Lavoisier in the late 18th century. Its properties as an energy source were soon recognized, culminating in the first hydrogen-filled balloon flight by Jacques Charles in 1783, demonstrating its practical utility.
The 20th century saw hydrogen revolutionizing industries. Its use in the Haber-Bosch process catalyzed agricultural productivity by enabling large-scale fertilizer production. During World War II, hydrogen played a role in military applications, such as synthetic fuel production. Later, the space race highlighted its potential as rocket fuel, with NASA adopting liquid hydrogen for its Saturn V rockets.
Hydrogen played a central role in the development of the hydrogen bomb, a weapon significantly more powerful than atomic bombs. Unlike fission-based bombs, which split heavy atomic nuclei, the hydrogen bomb relies on nuclear fusion—the same process that powers the sun. The bomb uses isotopes of hydrogen, such as deuterium and tritium, which are forced to fuse under immense pressure and heat generated by a primary fission explosion. This fusion reaction releases an enormous amount of energy, exponentially increasing the bomb’s destructive power. The first hydrogen bomb, codenamed “Ivy Mike,” was detonated by the United States in 1952, marking a pivotal escalation in the nuclear arms race during the Cold War.
The late 20th and early 21st centuries marked a shift toward hydrogen’s environmental promise. Concerns over fossil fuel emissions spurred innovation in hydrogen fuel cells, culminating in the development of hydrogen-powered vehicles and renewable energy integration. Today, hydrogen is a symbol of hope in the fight against climate change, with governments and private enterprises striving to unlock its potential.
Hydrogen fuel cells generate electricity through an electrochemical reaction between hydrogen and oxygen, producing water as the only byproduct. This clean energy technology is gaining traction as a solution to decarbonize transportation. Unlike conventional batteries, fuel cells offer faster refueling times and longer ranges, making them suitable for heavy-duty vehicles like trucks, buses, and trains. The future potential of hydrogen-powered vehicles lies in expanding green hydrogen production, improving fuel cell efficiency, and building a comprehensive refueling infrastructure. As governments and automakers invest in hydrogen technology, it could complement battery-electric vehicles and play a critical role in achieving net-zero transportation systems globally.
The journey of hydrogen – from a mysterious gas to a key enabler of a sustainable future – epitomizes humanity’s ability to harness nature’s elements for progress. Its history reflects not only scientific ingenuity but also the adaptability required to address evolving global challenges.
Download This Post in PDF
RESEARCH DISCLOSURE
Purpose: DUQUANT ANALYTICS (PTY) LTD provides research notes and newsletters for market insights, analysis, informational, educational, and research purposes only. We adhere to the CFA Institute’s Code of Ethics and Standards of Professional Conduct which includes maintaining the highest standards of integrity, transparency and professionalism in all research activities. We strive to ensure that our investment analysis and actions remain unbiased and focused on delivering accurate information based on available knowledge at the time of release. We follow industry accepted methodologies for conducting due diligence and conduct our analysis on a reasonable basis.
Investor Responsibility and Limitation of Liability: This content does not constitute a recommendation to buy or sell any securities. It is essential to understand the nature of our research, which is for educational and informative purposes. It is imperative to conduct your own due diligence and research prior to making any investment. DUQUANT ANALYTICS (PTY) LTD is not liable for any losses incurred from using our research. Information released is believed to be reliable, but not guaranteed for accuracy or completeness. It is also important to note that past performance is not reflective of future performance.
Potential Conflict of Interest: Personal Investments: Members of DUQUANT ANALYTICS (PTY) LTD may hold positions in some of the assets or securities mentioned in our research notes and newsletters. This includes equities, bonds, commodities and other financial instruments. Despite any personal investments, our research is conducted with the highest level of integrity, independence and objectivity. We strive to ensure that our analysis remains unbiased by focusing on accurate, public and provable information and secondary data as it is released or becomes public.
Conclusion: DUQUANT ANALYTICS (PTY) LTD is committed to providing high-quality research and insights to our readers. However, it is essential for investors to take responsibility for their own investment decisions and to understand the inherent risks involve, especially in mining which carries more risk than traditional assets. We encourage you to consult with a qualified financial advisor and to perform thorough research before making any investments.
Thank you for reading this disclosure note. We value your trust and strive to maintain the highest standards of integrity in all our research activities.