Navigating Uranium
With rising energy demand, decarbonization goals, and the resurgence of nuclear power, could uranium’s role in the global energy mix make it a critical investment opportunity?
Scientific Properties of Uranium
Uranium is a dense, silvery-grey metallic element with the atomic number 92, belonging to the actinide series. It is mildly radioactive and notable for its high atomic weight, with its most common isotopes being uranium-238 and uranium-235. Uranium-235 is fissile, meaning it can sustain a nuclear chain reaction, which is why it is key in nuclear energy and weaponry. A fascinating fact about uranium is its natural fluorescence; its compounds were used to create yellow and green glassware in the 19th and early 20th centuries. Uranium ore undergoes several refining steps, including crushing, leaching, and chemical precipitation, to produce uranium concentrate, commonly known as yellowcake. This bright yellow powder contains concentrated uranium oxides and serves as an intermediate product for further enrichment. Uranium is also the heaviest naturally occurring element on Earth that is still found in significant quantities.
Uses and Future Applications
Uranium’s primary use is as fuel in nuclear reactors, where it generates around 9% of the world’s electricity at ~439 operating nuclear reactors globally. These existing reactors make up a capacity of around 396GWe of electricity, and requires around 67,500 tonnes of uranium (tU) per year from mines to meet demand. Each additional GWe of new nuclear capacity will require roughly 300-450 tU for the first fuel load, and about 150 tU per year of additional uranium mine production thereafter to meet demand. The countries with the largest capacity are USA (~97GWe), France (~61.3GWe) and China (55.7GWe).
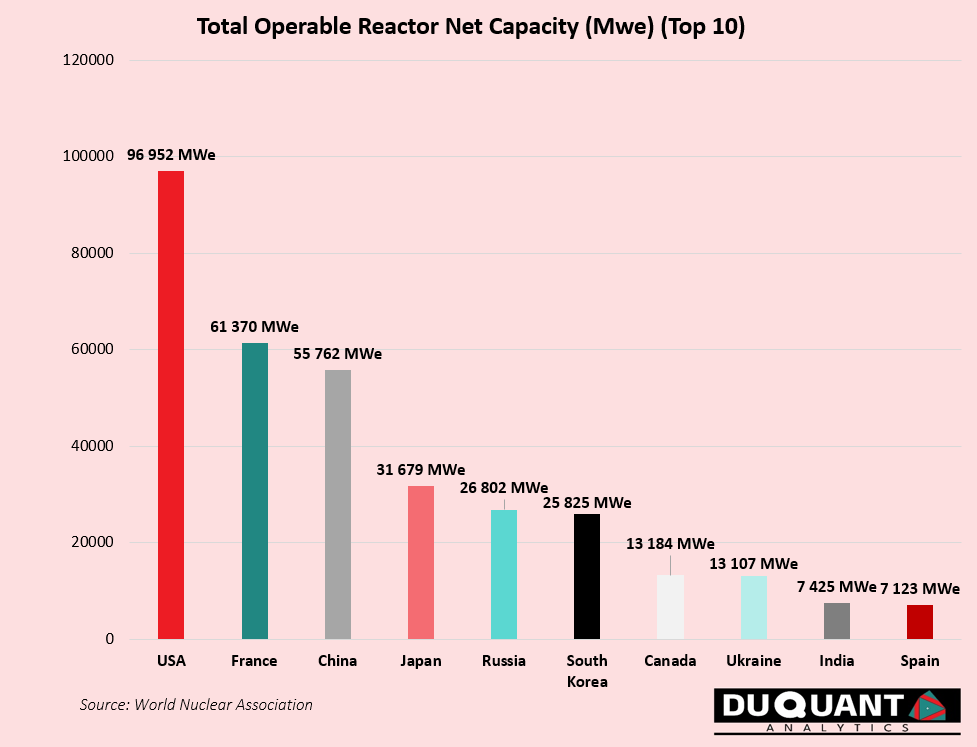
Nuclear energy within the global energy mix makes up around 9% of supply currently, and peaked in the 1990’s when it made up more than 16%. The growth in supply of cheap natural gas, as well as the growth of emerging markets that use more easily available electricity sources such as coal power plants, has lead to a decline of nuclear in the global electricity basket. However, due to global natural gas disruptions, and fluctuating oil and coal prices (highlighted in the 2022 energy crisis), plus complications of transporting natural gas, has been increasing the need to diversify the energy mix. Nuclear energies stability and long life has unique advantages in use cases to fill this need, especially in cases like data center’s that require uninterrupted electricity.
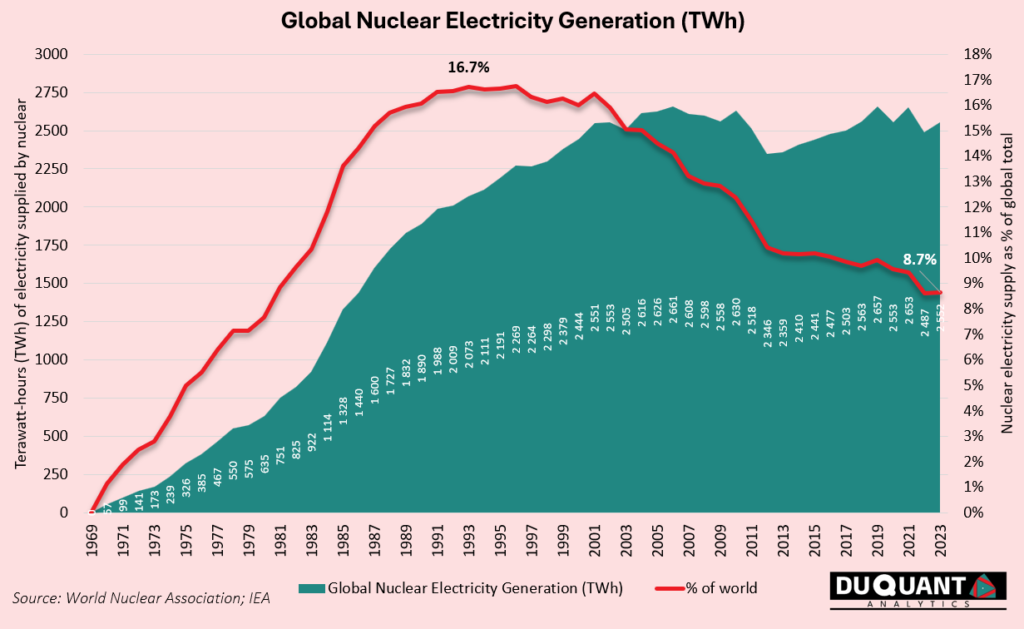
A single ton of uranium fuel pellets can produce about 45 million kilowatt-hours (MWh) of electricity, enough to power approximately 4,000 homes for a year. Inside a typical nuclear reactor, the fuel pellets are used for 3 to 5 years before they are replaced. However, not all the uranium is consumed; some remains as isotopes or waste products. The remarkable energy density of uranium means that a small amount of fuel provides an immense and consistent output, making nuclear power a highly efficient source of electricity. To match the energy output of 1 ton of uranium fuel, a solar farm would need to operate with peak efficiency for 25-30 years, assuming a capacity factor of about 20% (accounting for nighttime and weather). A solar farm covering hundreds of acres with tens of thousands of panels would be required to match the compact energy density of uranium. However, solar has advantages like lower waste generation and emissions but depends on intermittent sunlight, requiring battery storage or backup systems to achieve comparable reliability to nuclear power.
Uranium is also used in military applications, including nuclear submarines and nuclear weapons. Beyond energy, depleted uranium, a by-product of uranium enrichment, is used for its density in armour-piercing projectiles and radiation shielding. Looking ahead, uranium could play a pivotal role in advanced nuclear technologies, such as small modular reactors (SMRs) and fusion energy, both of which are seen as key to achieving net-zero emissions and have a host of use cases to power critical data centre infrastructure. These future applications, coupled with a growing global focus on clean energy, make uranium a compelling prospect for investors.
Largest Sources and Producers of Uranium
Uranium extraction from sandstone begins with mining, either by traditional open-pit/underground methods for higher-grade ores (typically 0.05% to 0.3% U₃O₈) or in-situ recovery (ISR) for lower-grade deposits. In ISR, solutions like sulphuric acid are injected into the sandstone to dissolve uranium, which is then pumped to the surface. The uranium-rich ore or solution is processed through crushing and leaching to dissolve uranium minerals, followed by purification via ion exchange or solvent extraction. The result is yellowcake (uranium oxide concentrate, ~80-90% U₃O₈). This yellowcake is then transported to a conversion facility for further refining. Uranium yellowcake (U₃O₈) is first converted into uranium hexafluoride (UF₆) through chemical processes, making it suitable for enrichment. In this step, the proportion of the fissile isotope U-235 is increased from 0.7% (natural levels) to 3-5% using methods like gas centrifugation. The enriched UF₆ is then converted into uranium dioxide (UO₂) powder, which is pressed into small cylindrical pellets and baked at high temperatures to create durable ceramic fuel. These pellets are loaded into zirconium alloy tubes to form fuel rods, which are bundled together into fuel assemblies used in nuclear reactors.
According to the World Nuclear Association, global uranium resources stand at just over 6 million tons U, with the largest resources being Australia (1684 Kt, or 28% of global), followed by Kazakhstan (815Kt or 13%), Canada (588.5 Kt, or 10%), Russia (480.9 Kt or 8%), Namibia (470 Kt or 8%), India (415.8 Kt or ~7%), South Africa (320.9 Kt or ~5%), Niger (311 Kt or ~5%) and Brazil (276.8 Kt or ~5%). The remainder of the world makes up only around 12% of global uranium reserves.
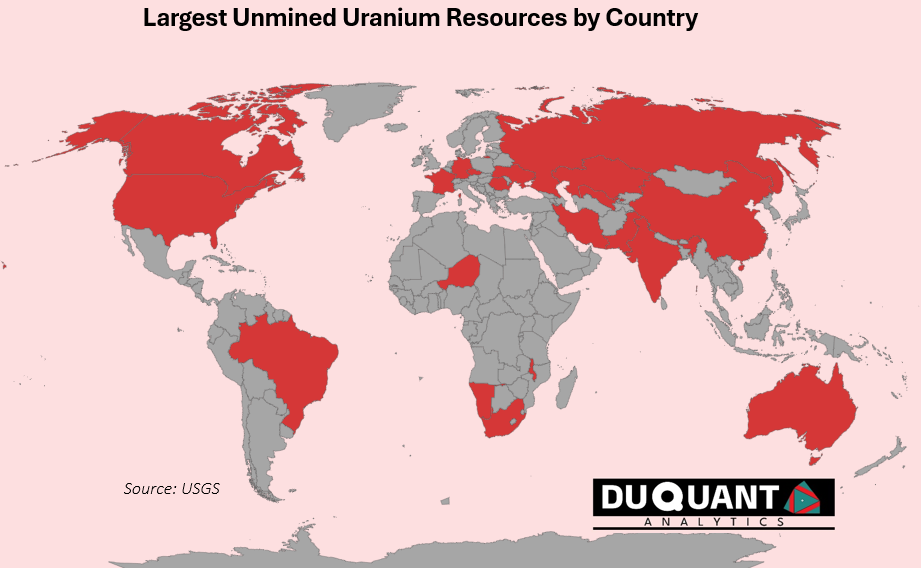
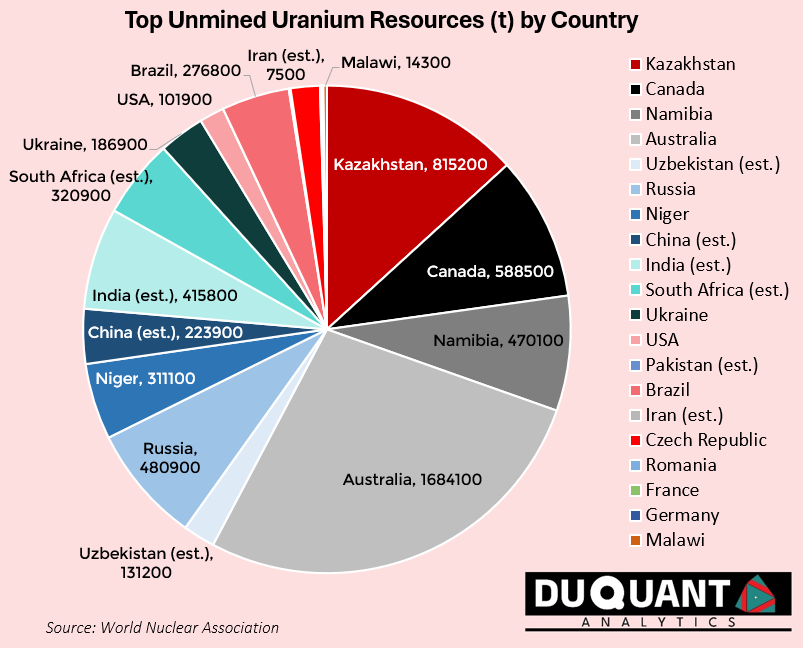
Uranium mine production has decreased at a CAGR of -2% over the last decade, from 59,331t in 2013 to an annual supply of 49,355t in 2022. Since 2013 approximately 41% of uranium came from Kazakhstan, followed by Canada (16%), Australia (10%), Namibia (8%), Uzbekistan (6%), Niger (6%), Russia (5%), and China (3%). The rest of the world has made up c.5% of the total mined supply of uranium since year 2013.
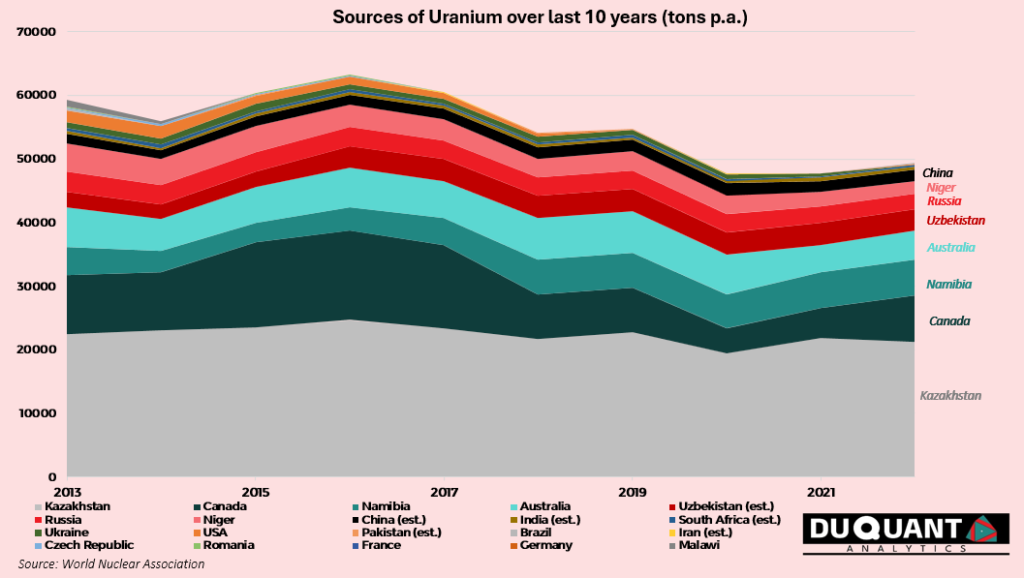
Based on the current annual production rate, and the estimated global resources, there is c.123 years remaining of uranium supply and thus could become a critical element in the energy mix given that it has more life than oil reserves of ~47 years supply remaining.
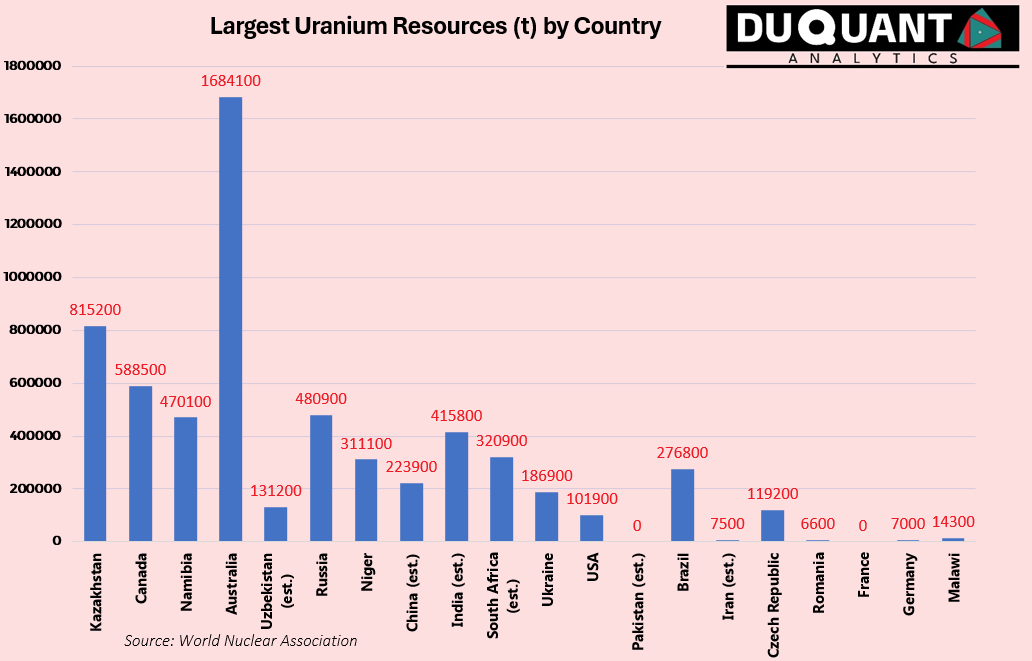
The Remarkable History of Uranium
Uranium’s story begins billions of years ago in the heart of dying stars, where its atoms were forged during supernova explosions. When Earth formed, uranium—along with other heavy elements—was incorporated into its crust. Early humans unwittingly encountered uranium in colourful minerals like pitchblende and carnotite, used for centuries to create vivid pigments in glass and ceramics. However, its true potential remained hidden until the dawn of modern science.
In 1789, German chemist Martin Heinrich Klaproth discovered uranium as a distinct element while analysing pitchblende. He named it after the recently discovered planet Uranus. For over a century, uranium’s primary use was decorative, admired for its yellow-green glow. Its radioactive nature was revealed in 1896 by physicist Henri Becquerel, who noticed it emitted energy spontaneously—an observation that laid the foundation for the field of nuclear physics.
In the early 20th century, Einstein entered the scene with his most famous contribution to nuclear science, — his equation E=mc², published in 1905 as part of his special theory of relativity. This equation revealed that mass and energy are interchangeable, providing the theoretical foundation for understanding how a small amount of matter, like uranium, could release an immense amount of energy during nuclear fission. While Einstein did not discover nuclear fission— uranium’s potential for energy was demonstrated through the discovery of nuclear fission by Otto Hahn and Fritz Strassmann in 1938. This breakthrough unleashed immense possibilities, but also immense dangers.
During World War II, uranium became central to the Manhattan Project, the secret U.S.-led effort to develop nuclear weapons. At its helm was J. Robert Oppenheimer, a theoretical physicist who served as the scientific director of the project. Oppenheimer coordinated the efforts of brilliant minds at Los Alamos Laboratory to harness the power of uranium and plutonium, leading to the creation of the first atomic bombs. The culmination of their work came in 1945, when the uranium-based bomb “Little Boy” was dropped on Hiroshima, Japan, followed by the plutonium-based bomb “Fat Man” on Nagasaki. These events marked a turning point in human history, demonstrating uranium’s devastating potential. After the war, Oppenheimer famously voiced his moral reservations, quoting the Bhagavad Gita: “Now I am become Death, the destroyer of worlds.”
In the post-war era, uranium’s role shifted toward peaceful applications (albeit, still considered controversial given accidents such as 2011 Fukushima). Uranium has become the cornerstone of nuclear energy, powering reactors that now supply up to 10% of the world’s electricity. Despite its promise, uranium remains a symbol of humanity’s dual-edged technological progress—a resource capable of both advancing civilisation and posing existential risks. Oppenheimer’s legacy and the story of uranium are inseparably intertwined, reflecting the immense power and responsibility that come with scientific discovery.
The future of nuclear energy holds promising applications in emerging fields such as data centre’s and artificial intelligence (AI). As global demand for computing power grows, data centre’s require vast amounts of electricity to support high-performance computing, especially for AI and machine learning tasks. Traditional power sources, while abundant, often struggle to provide the consistent, high-density energy required for these operations. Nuclear energy, with its reliable and scalable output, offers an attractive solution for powering data centre’s, especially in regions seeking to balance energy demand with sustainability goals. Small modular reactors (SMRs), a new development in nuclear technology, could provide a clean, safe, and compact energy source, reducing the carbon footprint of AI and big data operations. As AI systems become more energy-intensive, nuclear power could play a critical role in ensuring that the technological advancements driving these industries remain sustainable and low-carbon, supporting the growing demand for digital infrastructure.
Download This Post in PDF
RESEARCH DISCLOSURE
Purpose: DUQUANT ANALYTICS (PTY) LTD provides research notes and newsletters for market insights, analysis, informational, educational, and research purposes only. We adhere to the CFA Institute’s Code of Ethics and Standards of Professional Conduct which includes maintaining the highest standards of integrity, transparency and professionalism in all research activities. We strive to ensure that our investment analysis and actions remain unbiased and focused on delivering accurate information based on available knowledge at the time of release. We follow industry accepted methodologies for conducting due diligence and conduct our analysis on a reasonable basis.
Investor Responsibility and Limitation of Liability: This content does not constitute a recommendation to buy or sell any securities. It is essential to understand the nature of our research, which is for educational and informative purposes. It is imperative to conduct your own due diligence and research prior to making any investment. DUQUANT ANALYTICS (PTY) LTD is not liable for any losses incurred from using our research. Information released is believed to be reliable, but not guaranteed for accuracy or completeness. It is also important to note that past performance is not reflective of future performance.
Potential Conflict of Interest: Personal Investments: Members of DUQUANT ANALYTICS (PTY) LTD may hold positions in some of the assets or securities mentioned in our research notes and newsletters. This includes equities, bonds, commodities and other financial instruments. Despite any personal investments, our research is conducted with the highest level of integrity, independence and objectivity. We strive to ensure that our analysis remains unbiased by focusing on accurate, public and provable information and secondary data as it is released or becomes public.
Conclusion: DUQUANT ANALYTICS (PTY) LTD is committed to providing high-quality research and insights to our readers. However, it is essential for investors to take responsibility for their own investment decisions and to understand the inherent risks involve, especially in mining which carries more risk than traditional assets. We encourage you to consult with a qualified financial advisor and to perform thorough research before making any investments.
Thank you for reading this disclosure note. We value your trust and strive to maintain the highest standards of integrity in all our research activities.