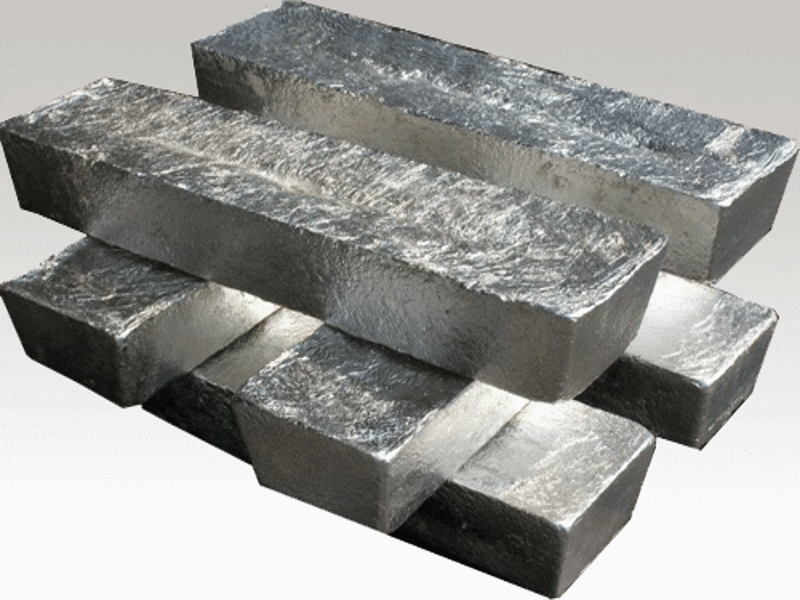
Navigating Manganese (Mn)
Scientific Properties of Manganese
Manganese is a transition metal with atomic number 25 and symbol Mn. Its atomic structure consists of 25 protons and typically 30 neutrons in the nucleus, surrounded by electrons distributed in various energy shells. Chemically, manganese exhibits multiple oxidation states, ranging from -3 to +7, with the most common states being +2, +3, +4, +6, and +7. This versatility allows manganese to participate in a wide range of chemical reactions and industrial processes. It has a relatively high melting point of 1246°C and a density of about 7.3 grams per cubic centimetre. In its pure form, manganese is silvery-grey in appearance, and while it is initially brittle, it can become ductile and malleable when properly heated and processed. These properties make manganese valuable in alloy production, particularly in steelmaking, where it enhances strength, hardness, and resistance to wear and corrosion.
Physically, manganese is typically found in a solid state at room temperature. It possesses paramagnetic properties, meaning it is weakly attracted to magnetic fields. This property finds application in various electronic and magnetic devices. Manganese is also a good conductor of electricity, contributing to its use in alloys and electrical components where conductivity is crucial. Its silvery-grey colour and metallic lustre make it recognizable in its pure form
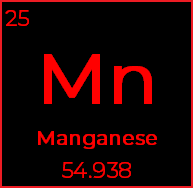
Uses and Future Applications
When looking at the critical metals and rare earth metals that will benefit from a green energy transition, bulk metals such as steel and manganese are often lost in translation and forgot about, and thus, seen as less attractive investments. This assumption can be far from true. Steel has built the entire developed world and economy as we know it, and will continue to do so as we evolve into a more sustainable economy. Each ton of steel contains about 4kg to 8kg of Manganese.
Globally, around 90% of manganese consumption is in the production of steel, and more importantly, construction-grade steel is only possible due to the inclusion of manganese. When producing steel, manganese is an essential component that improves strength, hardness, workability and long-term durability of the material. When looking at steel at large, it makes up the foundation of our entire modern society and is used in almost all buildings and modern infrastructure, as well as in transportation. Steels exists in buildings and skyscrapers, mobile network and telecoms towers, bridges, railways, equipment, and even in vehicles and ships. Demand for steel is likely to remain robust into the future, and growth is likely to be driven part by the industrialisation of emerging markets and infrastructure projects, as well as from a green energy transition. Solar panels and wind turbines for example, require steel support structures as a prerequisite for installation and proper functioning. Manganese is likely to benefit off the back of greater global demands for steel.
Manganese is also used in lithium-ion batteries where it helps to improve the battery stability and energy storage capacity. Manganese dioxide (MnO2), is also an essential component used as a cathode material in alkaline and zinc-carbon batteries. Manganese dioxide is a depolarizer and facilitates the conversion of chemical energy into electrical energy during energy discharge. Its stable chemical structure and high capacity for electron transfer make it ideal for enhancing battery performance, improving efficiency, and extending battery life in various applications from portable electronics to industrial equipment.
Manganese has extensive application across multiple sectors outside of steel production, such as in the chemical industry where manganese compounds play a critical role in the production of fertilizers, pigments, and ceramics. It is also a key element in alloy production, enhancing the properties of aluminium alloys through its desulfurizing and deoxidizing capabilities. Manganese is also utilized in electronics and agriculture where it serves as a vital micronutrient in the production of fertilizers and water treatment, where its oxidizing properties contribute to effective purification processes.
Largest Sources and Producers of Manganese
Manganese deposits are primarily categorized into three types: sedimentary, hydrothermal, and supergene. Sedimentary manganese deposits, the most significant source, form in shallow marine environments where manganese precipitates from seawater, often in association with iron. These deposits include manganese nodules found on the ocean floor and terrestrial deposits like those in South Africa’s Kalahari Manganese Field. Hydrothermal deposits occur near volcanic activity, where manganese is transported and deposited by hydrothermal fluids, typically found at mid-ocean ridges and geothermal areas. Supergene deposits result from the weathering of primary manganese-bearing rocks, where manganese is leached and re-precipitated closer to the Earth’s surface, forming high-grade manganese ores. Understanding these deposit types is crucial for exploration and mining, as each requires different extraction techniques and presents unique challenges and opportunities. The figure below highlights the locations around the world with significant manganese reserves.
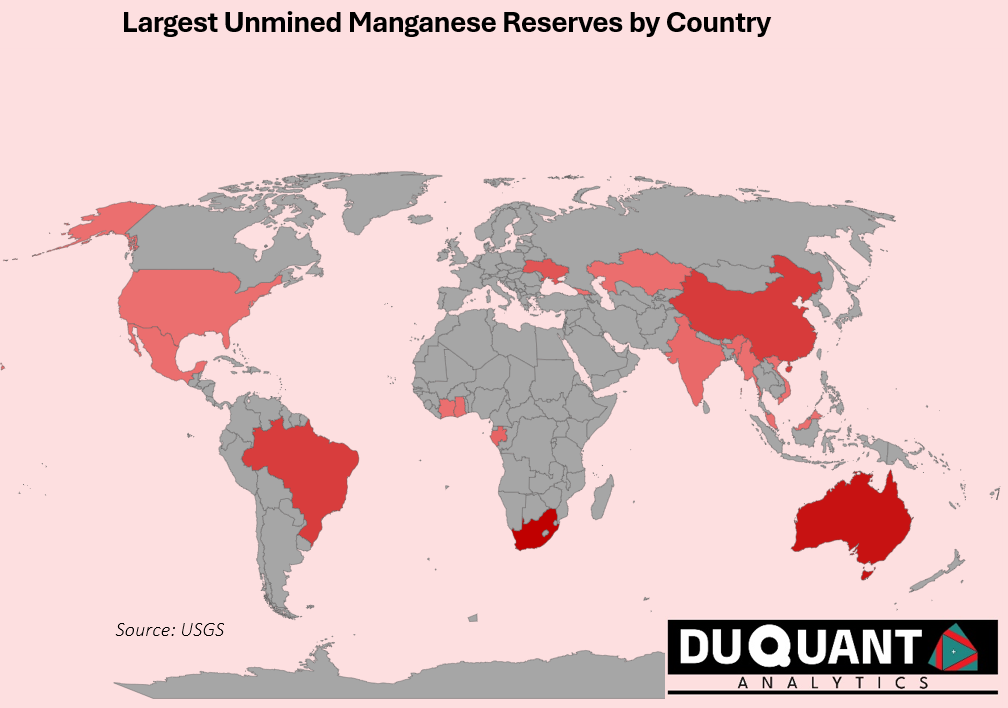
Current known reserves of manganese are highly concentrated in a few countries, which include: South Africa (32%), Australia (26%), China (15%), Brazil (14%) and Ukraine (7%). The rest of the world only makes up 6% of the remaining known reserves. The most significant discovery is the Kalahari Manganese Field in South Africa, is called “The BIG One,” and makes up 70%-80% of the worlds land-based manganese and was discovered in 1907. This allows for surface-level mining with reduced costs.
World manganese reserves according to the United States Geological Survey stand at 1,900,000 kilo tons (Kt or 1000t), with 600Kt from South Africa, 500Kt from Australia, 280Kt from China, 270Kt from Brazil, and 140Kt from Ukraine. These countries will be crucial to the supply of manganese, and thus steel production worldwide.
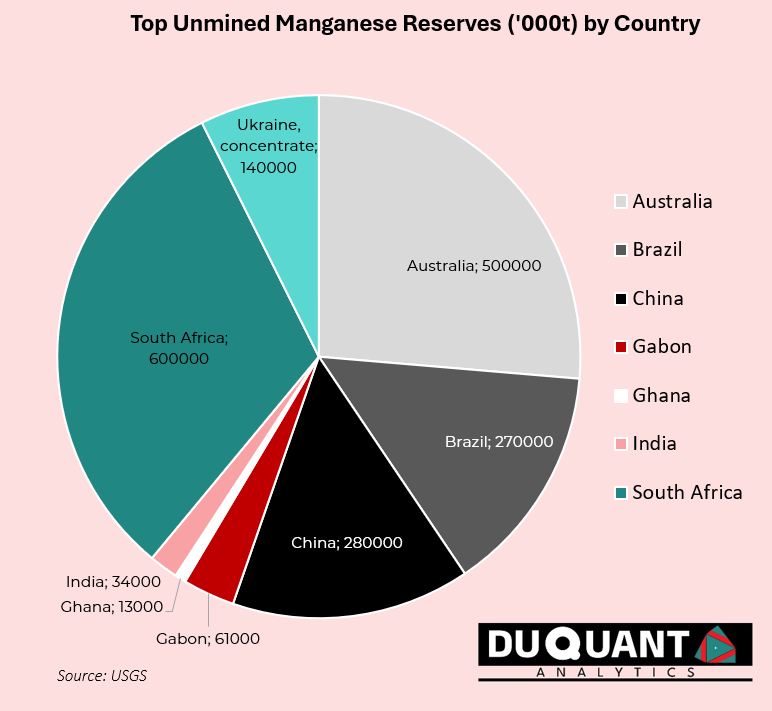
Manganese mine production has increased at a low CAGR of 1.1% from 2018 till 2023, with 34% of manganese since 2018 coming from South Africa, followed by Gabon (18%) and Australia (16%). Since the year 2000, approximately 27% of global production came from South Africa, 17% from Australia, 13% from Gabon, and 12% from China, – as seen below.
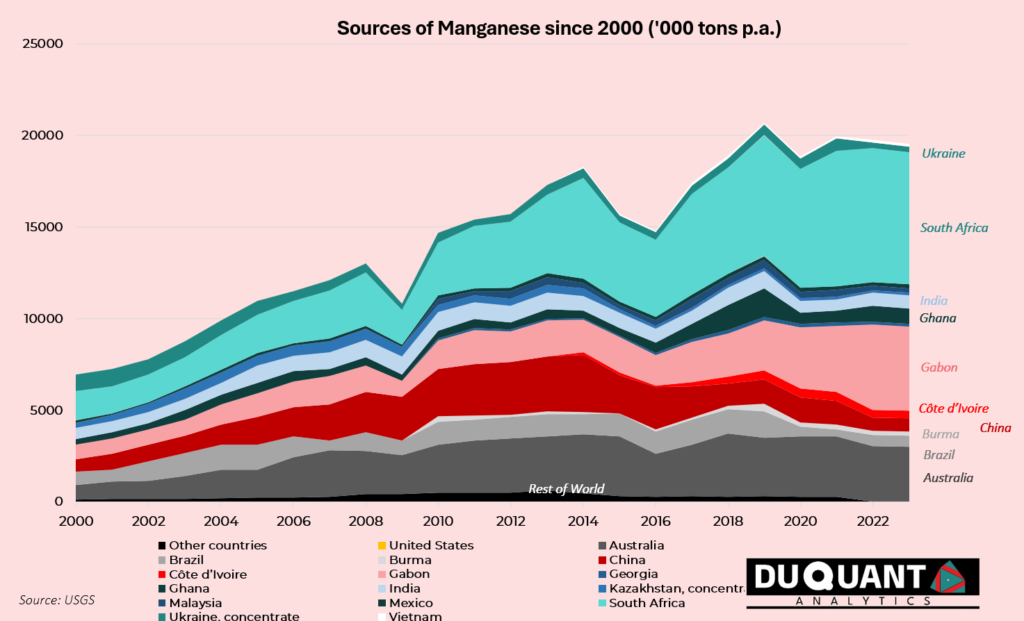
At current annual mine production rates, and known reserves, the world has c.95 years of manganese supply remaining. Although, it is estimated by Euro Manganese, that global manganese production will need to increase by 10X to meet demand requirements for a net zero transition. If this were to be the case, the world has less than 10 years remaining on manganese reserves in order to accommodate this increase in production. Further exploration will need to be established to expand known reserves.
The Remarkable History of Manganese
Manganese, an element crucial to steel production and modern technology, has a rich history intertwined with human advancement. Its journey from an obscure mineral to an essential component in industries worldwide is a fascinating tale of discovery, innovation, and adaptation.
Manganese’s story dates back to ancient times when it was used unknowingly in pigments and glassmaking. As early as 2,000 BCE, Egyptians and Romans used manganese dioxide to add colour to glass and pottery. The bright purple and black pigments produced by manganese compounds were highly prized, though the metal itself remained a mystery. In ancient Greece, manganese compounds were used in the production of iron and steel, enhancing their strength and durability. This practice laid the foundation for future metallurgical advancements, even though the Greeks did not fully understand the role manganese played in these processes.
The true nature of manganese was uncovered in the 18th century. In 1774, Swedish chemist Johan Gottlieb Gahn successfully isolated manganese metal by reducing its oxide with carbon. This discovery marked the beginning of manganese’s formal recognition as an element and its potential industrial applications. The 19th century witnessed the Industrial Revolution, which significantly increased the demand for manganese. Its essential role in steel production was discovered when it was found that adding manganese to iron removed impurities and improved the quality of the steel. This development was pivotal in the expansion of the steel industry, driving demand for manganese ore.
The early 20th century marked a period of rapid industrialization and technological advancement. Manganese became indispensable in steelmaking, used to deoxidize and desulfurize the metal, enhancing its strength and hardness. Major manganese deposits were discovered and mined in countries like India, Brazil, South Africa, and the Soviet Union, establishing them as key players in the global manganese market. During both World Wars, manganese was a critical resource for military production. It was essential for manufacturing high-strength steel used in weapons, vehicles, and infrastructure. The strategic importance of manganese led to increased mining activities and efforts to secure stable supplies.
The mid-20th century saw manganese’s applications expand beyond steel production. It became crucial in the production of batteries, particularly dry cell batteries, which used manganese dioxide as a depolarizer. This period also saw advancements in alloy production, with manganese being used to create aluminium alloys, enhancing their strength and resistance to corrosion. The late 20th century brought significant technological advancements that further increased manganese demand. The development of high-capacity batteries, such as alkaline and lithium-ion batteries, relied heavily on manganese compounds. These advancements propelled the metal into the spotlight, highlighting its critical role in modern technology.
Significant manganese deposits continued to be discovered and developed worldwide. South Africa emerged as a leading producer, with vast reserves in the Kalahari Manganese Field. Other notable producers included Australia, Gabon, and China, each contributing to the growing global supply. The early 2000s marked a significant increase in manganese demand due to the burgeoning battery market. The rise of portable electronics and electric vehicles (EVs) drove the need for high-performance batteries, leading to increased manganese mining and refining activities. The 2008 Global Financial Crisis highlighted the need for resilient and sustainable energy sources. Investments in renewable energy infrastructure and battery technologies surged, driving further demand for manganese. This period saw significant advancements in battery chemistry and increased production capabilities.
The 2010s brought increased scrutiny to the ethical sourcing of manganese, particularly from countries with less stringent environmental and labour regulations. Initiatives to improve mining practices and traceability became a focus for the industry, ensuring that manganese production met ethical and environmental standards. The 2020s have been characterized by an accelerated transition to renewable energy and electric transportation. Governments worldwide have implemented policies to promote EV adoption and reduce carbon emissions, further boosting manganese demand. This period has also seen advancements in battery recycling technologies to ensure a more sustainable supply of manganese.
With the rapid expansion of the manganese industry, environmental and ethical considerations have become paramount. The extraction of manganese, particularly in regions with rich deposits, poses significant environmental and social challenges. The industry has had to adapt by implementing more sustainable and ethical practices, investing in technologies to reduce environmental impact, and improving conditions for miners.
As the world continues to transition towards renewable energy and electrification, manganese’s importance is set to grow. The metal’s superior properties make it indispensable for next-generation energy storage solutions, including advanced batteries and energy-efficient technologies. Ongoing research aims to improve manganese extraction methods, enhance battery performance, and increase recycling rates. The future of manganese is closely tied to the global push for sustainability and energy efficiency. From its ancient use as a pigment to its central role in modern technology, manganese’s journey is a testament to its enduring value and versatility. Its story reflects the dynamic interplay between natural resources and technological advancements, shaping the future of our energy landscape.
Navigating Manganese
Navigating Manganese How is manganese poised to become the backbone of green tech, strengthening modern…